Liquids and Supercritical Fluids
The picture in the standard undergraduate textbook of the basic phases of matter on the pressure-temperature phase diagram is a relatively simple one (shown below). From the triple point onwards, the liquid state is separated from the solid state by the melting line and from the gas state by the boiling / vapour pressure line. This latter curve ends at the critical point, beyond which there is no transition between liquid and gas states; matter in this region of the phase diagram is in some halfway house between liquid and gas, known as the supercritical fluid state.
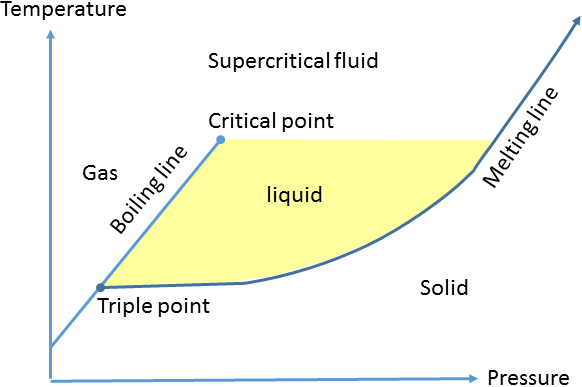
However, this simple model raises a number of questions. In the liquid fluid close to the melting line the sample has more in common with a solid than with a gas, in terms of density, heat capacity and viscosity for instance. So how does the fluid transition from the gas state, to a state with properties that have much in common with solids, when a path is followed going above the critical point so not encountering the boiling line (or any other first order phase transition)? Furthermore, the liquid state close to the melting line has characteristics that seem very similar to the supercritical fluid state close to the melting line, so is the critical temperature still a meaningful parameter at pressure / density significantly higher than the critical point?
It has been possible to study dense fluids with techniques such as X-ray and neutron diffraction, and Raman spectroscopy, and perform molecular dynamics simulations on these systems, for decades yet it is only recently (since 2012) that physicists have started to get at the answers to the questions above. Here at Salford we have been at the forefront of this research, producing key publications in Phys. Rev. E (2017) and J. Phys. Chem. Lett. (2019) and the first textbook to cover these recent advances.
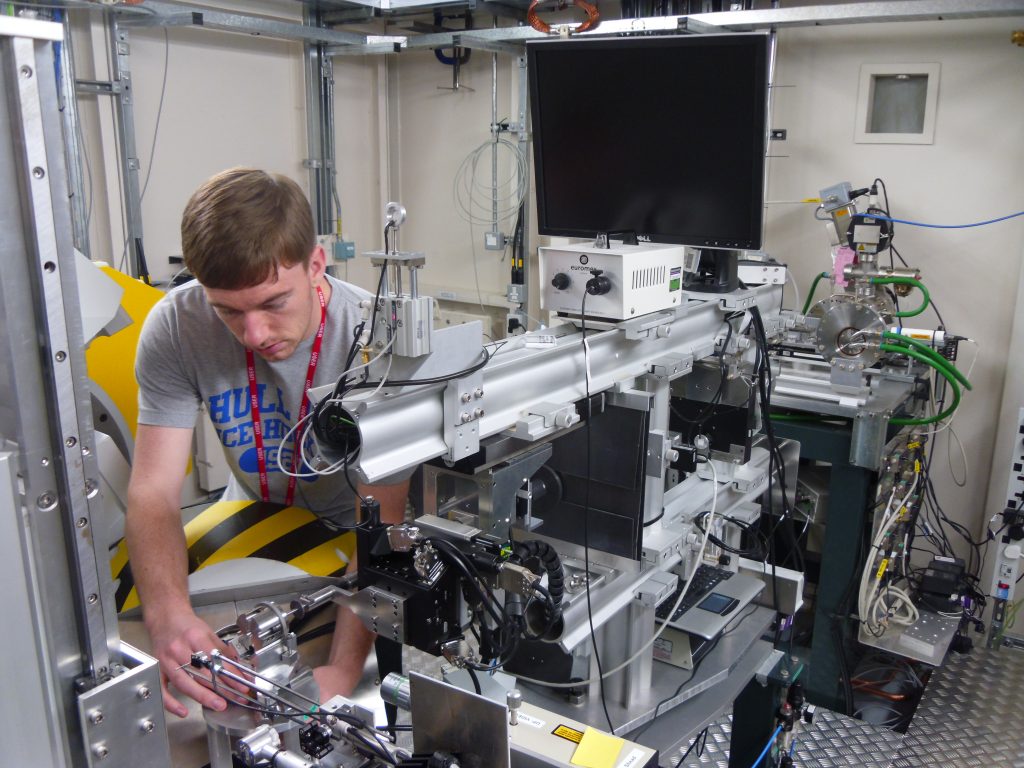
Experimentally we have conducted studies of dense liquids and supercritical fluids using our own facilities as well as at central facilities such as the Diamond Light Source, European Synchrotron Radiation Facility and ISIS Pulsed Neutron Source. Computationally we have performed molecular dynamics simulations at our own computing facilities, as well as developing our own code in C++ to test out the degree to which theoretical models based on resemblance to the solid state can explain the trends in heat capacity observed in dense liquids and supercritical fluids.
The modern picture of the liquid and supercritical fluid states is something like the diagram below, in which the liquid state is divided into regions exhibiting gas-like properties (non-rigid liquid), and properties reminiscent of a dense, rigid, liquid. The liquids we are familiar with in everyday life are generally rigid liquids.
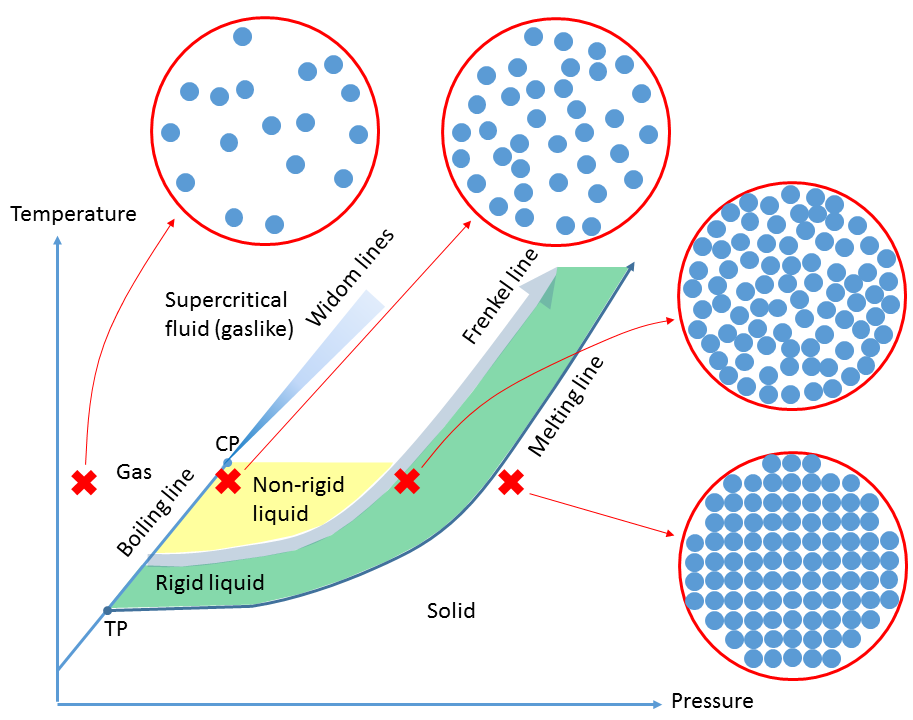
Unlike the non-rigid liquid, the rigid liquid can exist above the critical point up to arbitrarily high temperature if sufficient pressure is applied. The dividing line between these states (the Frenkel line, named after the Russian physicist Yakov Ilyich Frenkel) is not a discontinuous phase transition similar to melting or boiling lines, rather a narrow (but finite) region in which properties change from gaslike to liquidlike.
Having said that, the picture is constantly evolving. Very recently colleagues at the University of Edinburgh have used our neutron scattering data on fluid nitrogen to train their molecular dynamics simulations on this fluid, resulting in the prediction that the Frenkel line (instead of beginning somewhere on the boiling line) begins at the triple point.
Why is all this important? Well, supercritical fluids do have a range of industrial applications (reviewed in our recent book), but the main direct application of this work is in planetary science. Gas giant planets and many of their moons consist of complex fluid mixtures held under high pressure-temperature conditions at which the Frenkel line is likely to be encountered. Work to understand the consequences of the Frenkel line for the properties of fluid mixtures is currently at a very early stage. Our research on liquids and supercritical fluids continues, and postgraduate students have played a key role in this work over the years. If you are interested in applying, you can return to the homepage and read more on the postgraduate study menu, or start your application here.
Our recent publications on liquids and supercritical fluids
Structural Markers of the Frenkel Line in the Proximity of Widom Lines
C.G. Pruteanu, J.E. Proctor, O. Alderman and J.S. Loveday, J. Phys. Chem. B 125, 8902 (2021). Link
The liquid and supercritical fluid states of matter
JE Proctor, CRC Press (2020). Link
Modelling of liquid internal energy and heat capacity over a wide pressure-temperature range from first principles
J.E. Proctor, Phys. Fluids 32, 107105 (2020). Link
On the Transition from Gas-like to Liquid-like Behaviour in Supercritical N2
J.E. Proctor. C.G. Pruteanu, I. Morrison, I.F. Crowe and J.S. Loveday, J. Phys. Chem. Lett. 10, 6584 (2019). Link
Liquid Mysteries Physics World magazine, May 2018. https://physicsworld.com/a/liquid-mysteries/
Observation of liquid-liquid phase transitions in ethane at 300 K
J.E. Proctor, M. Bailey, I. Morrison, M.A. Hakeem and I.F. Crowe, J. Phys. Chem. B 122, 10172 (2018). Link
Dynamics, thermodynamics and structure of liquids and supercritical fluids: crossover at the Frenkel line
Yu.D. Fomin, V.N. Ryzhov, E.N. Tsiok, J.E. Proctor, C. Prescher, V.B. Prakapenka, K. Trachenko and V.V. Brazhkin, J. Phys.: Cond. Mat. 30, 134003 (2018). Link
Crossover between liquidlike and gaslike behaviour in CH4 at 400 K
D. Smith, M.A. Hakeem, P. Parisiades, H.E. Maynard-Casely, D. Foster, D. Eden, D.J. Bull, A.R.L. Marshall, A.M. Adawi, R. Howie, A. Sapelkin, V.V. Brazhkin and J.E. Proctor, Phys. Rev. E 96, 052113 (2017). Link